Project for Energetic Particle Chain
Energetic Particle Chain -Effects on the Middle/Lower Atmosphere from Energetic Particle Precipitations-
Energetic particle precipitation (EPP) due to solar activity occurs in the polar regions. The EPP particles create odd nitrogen (NOx) and odd hydrogen (HOx) which can affect the neutral chemistry of the middle atmosphere and impact the ozone concentration. However, so far, the observational data on these phenomena are quite limited. It is important to comprehensively understand the behavior of energetic particles in the magnetosphere, ionosphere, and atmosphere as a causal chain reaction system based on both observations and comprehensive simulations. The objectives of this project are to conduct multi-point and long-term observations using the Arase satellite, EISCAT_3D radar, riometers, and millimeter-wave spectroradiometers at the polar regions. These data will be used as inputs and constraints in modelling integrated simulation codes of EPP, ion chemistry in the atmosphere, and global dynamics/temperature field.
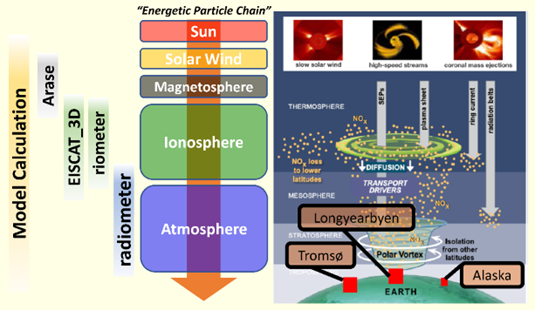
Left: Observational regions with each instrument and data fusion with simulation. Right (modified from Marshall et al., 2020): Relationship between the EPPs and new observation sites.